BIOCHEMISTRY TOPICS
Phosphorylated compounds in biochemistry
Phosphate and its acid-base chemistry. The phosphoryl group and examples of its occurrence.
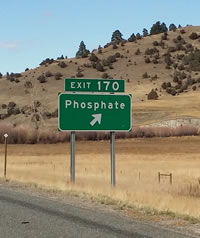
Phosphate is one of the most important inorganic ions in biochemistry. Phosphate plays a central role in the energetics of metabolism, and in biological structure and regulation as well. In in aqueous solution near physiological pH (7.0 - 7.5), inorganic phosphate (often abbreviated Pi and also sometimes called orthophosphate) exists primarily as H2PO4− or HPO42−, and these species constitute an effective buffer system in this pH range. Phosphate forms phosphoesters with the hydroxyl groups of a variety of biomolecules of all sizes, notably sugars (for example, glucose 6-phosphate, a phosphate ester of the 6-hydroxyl group of glucose) and proteins . This phosphorylation of the free hydroxyl via esterification adds negative charge to its target, which can markedly modify its properties. A phosphate with two ester linkages is called a phosphodiester, and this chemical motif is seen in both the repeated sugar-phosphate linkages forming the covalent backbone of nucleic acids and in cyclic nucleotides such as 3′,5′-cyclic AMP (cAMP). Phosphate is also incorporated into the polar head groups of phospholipids. The metabolically significant pyrophosphate is an anhydride formed between two phosphates, and is less thermodynamically stable than a phosphate ester. Thus, a molecule with a phosphoric anhydride linkage is relatively high-energy, and such a phosphate can be readily transferred to a free hydroxyl. (This type of phosphoryl transfer reaction is an important reaction that is catalyzed by enzymes called kinases.) The important carrier of metabolic chemical energy adenosine triphosphate (ATP) has two such high-energy phosphoric anhydride bonds. The activity of kinases are opposed by the that of phosphatases, which catalyze the hydrolysis of phosphate esters.
Phosphate acid-base chemistry
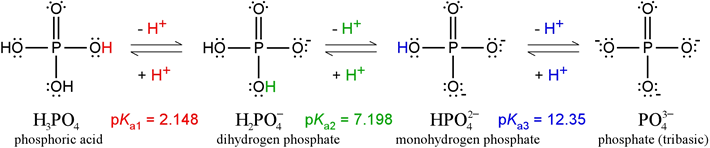
Phosphate, with its four distinct protonation/charge states, constitutes a triprotic system. The fully protonated form of phosphate is phosphoric acid (formula mass 98.00). Strictly speaking, the term "phosphate" refers to the fully deprotonated form. Neither of these forms exists in any significant quantity at or near physiological pH. The figure below illustrates all four of the species (in full Lewis structure representations, showing nonbonding electrons as well as covalent bonds). The predominant forms between pH 4 and 10 are the two intermediates, dihydrogen phosphate (monobasic) and monohydrogen phosphate (dibasic). Phosphoric acid is a relatively strong acid compared with most organic acids. Although the figure above shows one proton (in red) dissociating from phosphoric acid to form its conjugate base, dihydrogen phosphate, it should be clear that any of the three protons can dissociate, yielding the same conjugate base. This is one factor making phosphoric acid a better acid; another factor is resonance stabilization of the conjugate base. Only one of two possible resonance structures are drawn above for dihydrogen phosphate.
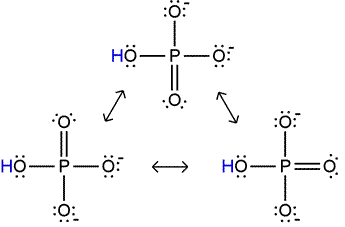
Dihydrogen phosphate itself can act as an acid - there are two protons that can dissociate, and its conjugate base, monohydrogen phosphate, is also stabilized by additional resonance structures (illustrated at left). However, the fact that a proton must dissociate in this case from a polyatomic anion, which is unfavorable because of the electrostatic energy required to separate positive and negative charges, makes dihydrogen phosphate a much weaker acid than phosphoric acid. Similar considerations apply to monohydrogen phosphate. Its double negative charge makes it a very weak acid, although its conjugate base, phosphate, can be represented by four resonance structures.
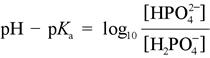
The Henderson-Hasselbalch equation can be applied to tell us the proportions of dihydrogen phosphate and monohydrogen phosphate present in a buffered system between pH 4 and 10. For instance, at pH = pKa of dihydrogen phosphate, the amounts of dihydrogen and monohydrogen forms are exactly the same. The concentrations of phosphoric acid and tribasic phosphate are well below negligible, and the average charge of phosphate species in solution (abbreviated by biochemists as Pi) is −1.5. As the pH increases above 7.2, the monohydrogen form increases at the expense of the dihydrogen form, and the average charge of phosphate species becomes more negative, approaching −2.
Representation of phosphate in biochemistry
As phosphate and phosphorylated compounds are ubiquitous in biochemistry, shorthand structural notation is commonly used, and this is illustrated in the figure below.
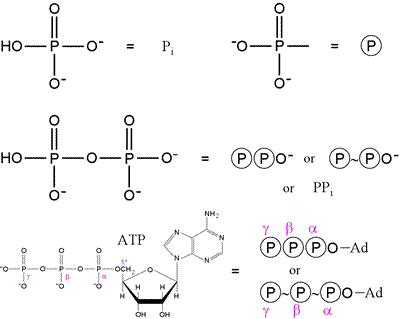
The first row shows that "inorganic" phosphate is represented by Pi, while a phosphoryl group or substituent on a molecule is often represented by the circle-P notation. On the second row, pyrophosphate (phosphoric anhydride) and its symbolic representation are shown. Finally, the ATP molecule can be represented in abbreviated form as shown, where Ad represents adenosine. Note the use of the "~", or "squiggle", notation, which indicates a place in the molecule where a reaction can release a significant amount of energy. Here, we see this high energy content - or, more precisely, phosphoryl transfer potential, associated with the anhydride type linkages seen in pyrophosphate and ATP. There are other compounds with higher phosphoryl transfer potential than ATP, for instance the glycolytic intermediate phosphoenolpyruvate (PEP). The squiggle notation, introduced by Fritz Lipmann in the 1940s, is not as commonly used today.
Phosphorylation
As noted above, phosphorylation is the attachment of phosphate to functional groups of a variety of biomolecules. Most commonly, phosphorylation involves the transfer of phosphoryl group from a phosphorylated donor compound to hydroxyl groups of target molecules. Phosphorylation of a free hydroxyl via esterification adds negative charge to its target. As the second pKa of a phosphate ester is typically 1 or more pH units less than pKa2 for phosphate, a −2 charge state predominates in the usual physiological pH region. The marked difference in properties between a phosphorylated and an unph osphorylated hydroxyl group has important functional effects in regulation of biological activity of the molecules undergoing such modification. Phosphorylation of biological molecules using nucleoside triphosphates such as ATP is catalyzed by enzymes called kinases. Removal of phosphates by hydrolysis is catalyzed by phosphatases. In many signal transduction pathways, regulated phosphorylation and dephosphorylation of signaling molecules plays a key role, and is reiterated in sequential cascades within these pathways. When proteins are targets for phosphorylation, the corresponding enzymes that catalyze these phosphorylations are called protein kinases. A well known example is protein kinase A. Most protein kinases catalyze the transfer of phosphate groups to specific serine, threonine, or tyrosine residues. Phosphorylations of other amino acid residues occur in some cases.
A classification of reactions involving phosphoryl or phosphate transfer can be made as follows (see Walsh, 1979):
- Transfer of phosphoryl groups between nucleophilic functional groups with oxygen or nitrogen atoms (kinases)
- Transfer of phosphoryl group from functional group (e.g. hydroxyl) to water (phosphatases)
- Transfer of phosphoester from phosphodiester to water (phosphodiesterases)
- Group transfer to phosphate (or phosphoester)- phosphorolysis.
Examples:
- Hexokinase [EC 2.7.1.1], protein kinase A [EC 2.7.11.11]; In phosphoenolpyruvate (PEP), phosphate is esterified to the enol form of pyruvate. This phosphoryl group can be exergonically transferred to ADP in a substrate-level phosphorylation reaction catalyzed by the enzyme pyruvate kinase [EC 2.7.1.40].
- Glucose 6-phosphatase [EC 3.1.3.9], phosphoprotein phosphatase [EC 3.1.3.16];
- cAMP phosphodiesterase [EC 3.1.4.17]
- Glycogen phosphorylase [EC 2.4.1.1]
See the glycolysis topics page for full discussion.
Related topics pages: