Lecture 1. Foundations
Introduction to the course. Inventory of concepts from prior courses that are important for biochemistry. Cellular foundations. The domains of life. Energy sources utilized by living organisms. Organization of cells. Supramolecular structures and eukaryotic organelles. Chemical foundations. Carbon-based biomolecules small and large (macromolecules), three-dimensional structures of biomolecules and their importance in determining biological function or effect. Physical foundations. Chemical thermodynamics and its relevance to biochemistry. Energy transductions in living organisms. Metabolism in biochemical systems.
Reading: Lehninger, 7th ed. ("Lehninger"); Ch. 1, pp.1-29.
Summary
We begin our semester-long journey exploring the chemistry of life by taking an inventory of the concepts and principles developed in the biology and chemistry courses you've taken up to this point. The list of courses we developed includes General Chemistry [CHEM 101], Organic Chemistry I & II [CHEM 230, 231], Information Flow in Biological Systems [BIOL 105], Energy flow in Biological Systems [BIOL 106], Genetics [BIOL 207]. Note that many of the concepts we'll find relevant are reviewed in the reading assignment (Chs. 1 & 2 of Lehninger).
We begin the material of the present course per se in the readings with setting a proper celluar and macroevolutionary context. We'll then turn to chemical foundations, developing a chemical description of biomolecules, including detailed attention to sterochemical and structural features. Finally, we'll undertake a review of thermodynamic principles, including forms of energy, the nature of entropy, and the laws of thermodynamics. We define spontaneous processes, free energy and its relationship to chemical equilibrium, and biochemical standard state.
The cellular nature of life
Cells are spatially defined as membrane-bounded microsystems of great functional and compositional complexity. They are thermodynamically open systems that are able to sustain a dynamic, non-equilibrium steady-state. Furthermore, cells have the ability to self-replicate, by mitosis, and form higher order associations as multicellular organisms. The internal environment of cells is regulated, and levels of ions, metabolites, chemical energy, reducing potential, and transmembrane electrochemical potential, are maintained within typically narrow ranges. This characteristic of cells, referred to as cellular homeostasis is apparently for their continued survival. One of the central tasks that concerns biochemistry is a complete molecular-level description of the mechanisms underlying homeostasis and self-replication.
Among the topics where there is a large intersection between cell biology and biochemistry are
- Compartmentation, catalysts, precursors, energy sources
- The distinction between prokaryotes and eukaryotes; eukaryotic organelles
- Prokaryotes (bacteria and archaea)
lack a nucleus or other membrane-bound organelles - Eukaryotes have specialized membrane-bound organelles: e.g. mitochondria, chloroplasts
- Prokaryotes (bacteria and archaea)
- Mechanical properties of cells and their ability to generate kinetic energy
- Cytoskeletal structure
- Motility: bacterial flagella
- Muscle contraction: myofibrils
Chemical foundations
The following important chemical concepts are foundational for biochemistry, in the
sense of constituting prerequisite knowledge.
- Aqueous solutions
- Properties of water as a solvent
- Aqueous chemistry:
acid-base reactions
- Definitions of pH, pKa, etc.
- Buffers and the Henderson-Hasselbalch equation
- Functional groups
- It is important to know the common functional groups from organic chemistry, their properties and reactivities (for a list, see Fig. 1.17, p.14 in the text).
- Examples of ionizable functional groups (carboxyl/carboxylate and protonated amine/amine conjugate pairs) with pH-dependent charge states. We will not memorize pK values, but it is important to have a "ballpark" idea of the values and know the order of acid strength of the various acidic functional groups.
- Nomenclature
- Redox reactions
- Oxidation-reduction, or "redox", reactions figure prominently in metabolism. An example is provided by the electron transport protein cytochrome c.
- Chemical kinetics: The velocity of chemical reactions
- Enzyme kinetics: Enzymes are biological catalysts that greatly speed up the rates of specific spontaneous chemical reactions.
- Thermodynamics
- Living organisms obey the laws of thermodynamics!
- A spontaneous process is one that is thermodynamically favored; however, the thermodynamic favorability of a process does not imply anything about its rate (kinetics).
- For processes occurring in a system at constant temperature and pressure,
the Gibbs free energy
function (G) is used to assess spontaneity:
- ΔG < 0 for the process ⇔ process is spontaneous
- In contrast to a state of chemical equilibrium, living organisms maintain a nonequilibrium steady state by utilizing energy sources (chemical potential and electromagnetic radiation).
- Covalent (bonding) interactions and noncovalent interactions
- It is quite important to understand the distinction between covalent or ionic bonding and noncovalent forces.
- Noncovalent forces determine the conformations (and three-dimensional shapes) of biological macromolecules and the interactions between molecules (see below for a summary of noncovalent forces)
The major classes of biological molecules
The major classes of biological molecules are proteins, nucleic acids, carbohydrates, and lipids. These molecules are characteristically very large - much larger than the "small" molecules we are used to dealing with in introductory chemistry courses. Thus, we often refer to these as biological macromolecules, which commonly have molecular masses in the range several thousand on up to millions of atomic mass units (amu). In biochemistry, instead of the amu, the equivalent unit dalton (D or Da) is used. The molecular weights of biological macromolecules are most conveniently expressed in kilodaltons (kD). Our initial goal is to learn the basic covalent structures of these classes of molecules, and susequently relate them to some of their properties or functions. For example, we'll repeatedly consider the class of biological macromolecules known as proteins, which are polymers of amino acids covalently linked together in a specific sequence by amide bonds. These linkages are given the special name peptide bonds, and the chains of amino acids that make up proteins are referred to as polypeptide chains or polypeptides.
A brief review of chemical thermodynamics
Biological systems are subject to the same physicochemical laws that govern non-living systems. It is important therefore to understand explicitly how basic thermodynamic principles are applied in biochemistry and biology. Therefore we undertake a review of the thermodynamics appropriate for application to biological systems. For example, we can usually treat processes in living organisms and tissues as occurring at constant pressure and temperature, with little or no change in volume. Thermodynamic methods valid in general for such processes - such as the Gibbs free energy function (G) - will be useful in this specific biological context.
The first law of thermodynamics is equivalent to the law of conservation of energy. For example, in a process occurring at constant pressure and volume involving exchange of heat between a system and its surroundings, the enthalpy change (ΔH) for the system will be exactly balanced by ΔH for the surroundings so that the net ΔH is zero. Conservation of energy applies to all processes, but says nothing about the direction that a process obeying it will operate in. A further aim of thermodynamics is to be able to predict whether or not a process will occur within a system without the input of energy from the surroundings. If so, the process is said to be spontaneous. In order to make such predictions, it is first necessary to define entropy, S. Roughly speaking, entropy is a measure of the number of ways that energy can be distributed within a system. The second law of thermodynamics states that the total entropy of the system and surroundings always increases for a spontaneous process.
For biochemical systems, it would be convenient to have a means to evaluate whether or not a process is spontaneous without having to consider the surroundings. The state function known as Gibbs free energy, G (sometimes referred to simply as free energy), which is defined by the equation G = H − TS that combines the state functions enthalpy and entropy (T is the temperature in K), is the key quantity that allows us to make this determination. The change in free energy of a system for a process occurring at constant temperature is given as ΔG = ΔH − TΔS. A spontaneous process corresponds to a decrease in the free energy of the system.
Key thermodynamic equations for biochemistry
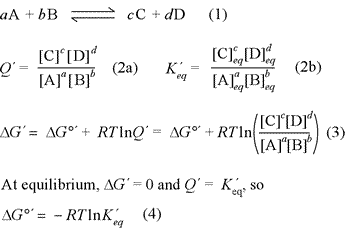
The relationship between free energy and chemical equilibrium is one of the most important results of chemical thermodynamics, and it retains this status in biochemistry. The equations at right, (1) - (4), are the key thermodynamic relations that are essential for you to know. The equations are general in form, so it is also necessary to understand how they are used in specific examples. The quantity ΔG°′ is the biochemical standard free energy change, which is based on the definition of a biochemical standard state. In all these relations, the prime [′] denotes biochemical standard conventions in order to clearly distinguish from chemical convention (physical chemistry standard conventions) for which all the relations above are the same, sans prime symbol. The key distinction to bear in mind for these conventions is for the standard hydrogen or hydronium ion concentration. While the physical chemistry standard [H+] is 1 M (just like for any solute species), in the biochemical convention the standard [H+] is specifically defined as 1 × 10−7 M, or pH 7.
Metabolism in biochemical systems
Metabolism is the entire set of chemical transformations carried out by living cells, tissues, and whole organisms that interconvert molecules called metabolites. As noted above, living organisms are capable of (and their existence derives and depends upon) generation of nonequilibrium steady states from exogenous energy sources. As biochemical systems, this entails a flow of matter through the system, denoted as flux. Metabolic flux is the flow of matter directed through a given reaction, or sequences of reactions. Certain sequences of reactions that interconnect central or terminal metabolites constitute named biochemical pathways. Our principal example in this course of such a metabolic pathway is glycolysis. Central roles in metabolism are performed by ATP and NAD(P)H. Feedback inhibition. Systems biology.