Lecture 5. Amino acids and multiprotic species
Tuesday 29 January 2019
Polyprotic species. pH calculations, titrations, and principal species analysis for diprotic and triprotic cases. The general structure and set of 20 "standard" α-amino acids. Assignment of configuration to chiral centers: Relative and absolute. Chiral side chains. Some notable features of selected amino acids: Histidine - an "ambidextrous" amino acid residue. Perturbed or "anomalous" pKa values.
Reading: Lehninger - Ch.3, pp.75-85.
Summary
Reading summary.
§3.1 Amino acids.
Amino acids share common structural features.
The amino acid residues in proteins are L stereoisomers.
Amino acids can be classified by R group.
Box 3-1 -Methods- Absorption of light by molecules: The Lambert-Beer Law.
Uncommon amino acids also have important functions.
Amino acids can act as acids and bases.
Amino acids have characteristic titration curves.
Titration curves predict the electric charge of amino acids.
Amino acids differ in their acid-base properties.
***
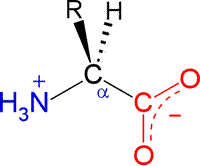
The general structural formula for an α-amino acid, shown at right. A central carbon atom is designated as C-alpha (Cα). Its constituent functional groups are the amino group (shown in blue) and the carboxylate group (shown in red). Note that these groups are shown according to their expected ionization state at a physiological pH of about 7. The amino group is accordingly protonated with its nitrogen bearing a positive formal charge. The carboxylate (often represented in abbreviated form on one line as COO−) is the principal species of a carboxylic acid functional group when pH > pKa.
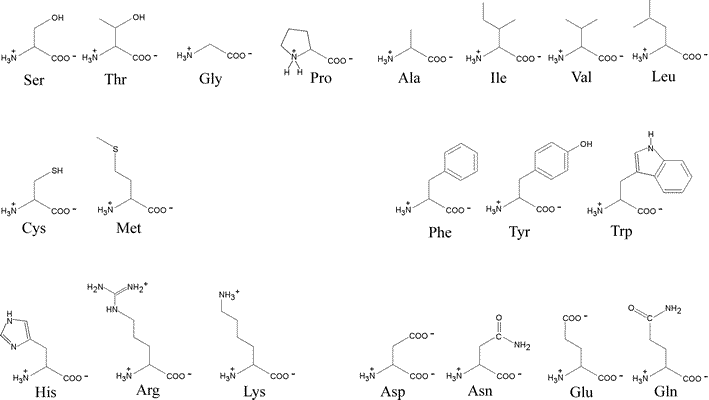
By convention, a polypeptide sequence can be represented by the single letter symbol for an amino acid, with the first letter listed the amino end or at the N-terminus and the last letter listed the carboxy end or C-terminus.
Acid-base chemistry of amino acids
The amino acids generally form diprotic or triprotic systems in aqueous solution. The figure below illustrates how the charge on a diprotic species (corresponding to an amino acid with an unionizable R group) varies with the "ambient" pH of the medium of which it is a component. The three possible species are positively-charged fully protonated form on the left, the intermediate form, which is a zwitterion, in the middle, and finally the fully deprotonated, negatively charged form on the right. In each of the three pH regions, 1 - 3, the species shown will be the principal (if not the only) species present. Of course, when pH = Ka1, the amounts of the fully protonated species and the intermediate, zwitterionic species will be equal. Similarly, when pH = Ka2, the amounts of the intermediate and fully deprotonated forms will be equal.
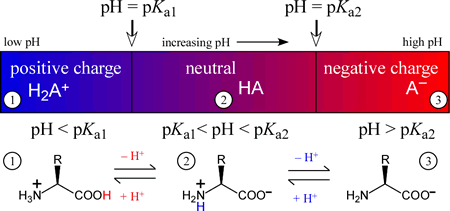
Histidine
Among the amino acids, histidine (His, H) is one of the most interesting because of the variety of roles it can play in protein function, especially as a key residue in many enzyme active sites. Of all the ionizable side chains, the typical pKa of the imidazole ring of His is closest to a neutral pH. Studies of model compounds have established a range of 6.0 - 7.0 for the intrinsic pKa of the histidine side chain.
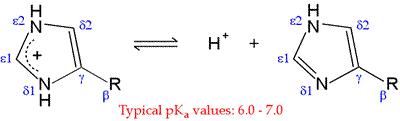
The neutral form of the imidazole ring can exist in two different tautomeric forms: with hydrogen on the δ1 nitrogen or with hydrogen on the ε2 nitrogen. The pKa of the ε2 nitrogen has been shown in 13-C NMR studies of a model compound to be about 0.6 pH units higher than that of the δ1 nitrogen, so in the absence of countervailing environmental effects, the form on the right will tend to predominate.
Perturbed or "anomalous" pKa values
As has already been suggested, the intrinsic pKa values for ionizable groups are no guarantee that a particular residue in a particular protein will be in a particular ionization state at a pH consistent with its physiologically relevant structure and function. Some pKa values are perturbed significantly from their intrinsic values, and these "anomalous" values are furthermore demonstrably important for the proper function of a protein in some cases.
To illustrate the idea, consider an aspartate residue in a neutral (pH 7) aqueous environment with a "normal" pKa. The residue will be overwhelmingly ionized. By plugging in values for the pKa of the residue, the pH of the medium, the Henderson-Hasselbalch equation can be used to calculate the proportion of ionized to unionized forms of the residue. The top half of the figure below illustrates the situation.
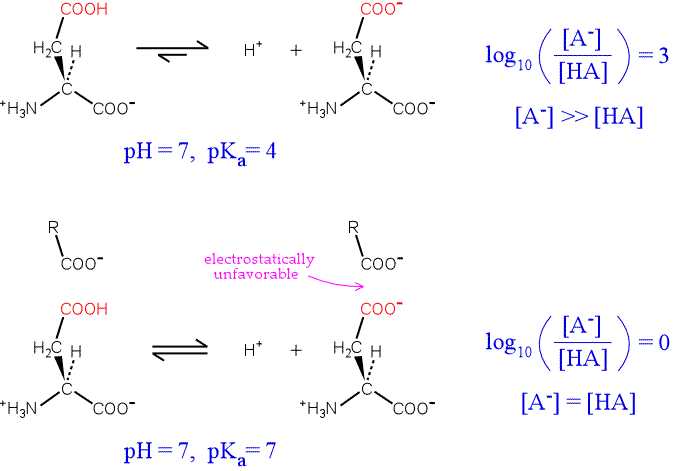
Now consider the influence of a nearby negative charge on the ionization of our hypothetical residue. The presence of the negative charge makes the ionization much less favorable, shifting the equilibrium to the left. The greater the shift in the equilibrium, the more the pKa is raised from its intrinsic value. In the extreme case illustrated, the amounts of both forms of the residue are equal, and the pKa has been perturbed upward by three units.
An example of this effect where the residue with an anomalous pKa is directly involved in the protein's function is provided by lysozyme. Lysozyme is an enzyme produced by a variety of organisms that hydrolyzes the polysaccharide component of the peptidoglycan cell walls of many types of bacteria. The mechanism of lysozyme depends on two acidic residues, Asp52 and Glu35. Asp52 has a normal pKa. Its negative charge stabilizes a developing positive charge as the reaction proceeds through a oxonium ion intermediate. However, Glu35 has an anomalously high pKa (its pKa is thought to be about 6.5), keeping it more in the protonated form. The unionized form of Glu35 donates a hydrogen ion to the oxygen of the glycosidic linkage, assisting the breaking of the bond between sugar residues. Glu35 would not be so effective in this role if its pKa was normal.