Lecture 13. Enzyme mechanism
Tuesday 5 March 2019
Enzyme mechanism. Catalytic principles and case studies of enzymes.
Reading: Lehninger - Ch.6, pp.213-225.
Summary
Reading summary. §6.4 Examples of enzymatic reactions. The chymotrypsin mechanism involves acylation and deacylation of a Ser residue. An understanding of protease mechanisms leads to new treatments for HIV infections. Interlude: How to read enzyme mechanisms--A refresher, plus Mechanism Figure 6-23, Hydrolytic cleavage of a peptide bond by chymotrypsin (pp.216-217). Hexokinase undergoes induced fit on substrate binding. The enolase reaction mechanism requires metal ions. Mechanism Figure 6-27: Two-step reaction catalyzed by enolase. Lysozyme uses two successive nucleophilic displacement reactions. Fig.6-28: HEWL and the reaction it catalyzes. Mechanism Figure 6-29: Lysozyme reaction (p.222). An understanding of enzyme mechanism produces useful antibiotics. Fig.6-30: The transpeptidase reaction (p.224). Fig.6-31: Transpeptidase inhibited by β-lactam antibiotics (p.224). Fig.6-32: β-lactamases and β-lactamase inhibition (p.225).
***
To illuminate general properties of enzymes, it is useful to examine in detail a few specific case studies of enzymes.
It may be useful at this point to review the Enzymology: An introduction topics page.
Lysozyme
Lysozyme constitutes a good example illustrating the role and importance of binding energy in enzymatic catalysis. A 14.6-kD globular protein discovered by Alexander Fleming in 1922. The bactericidal effect observed by Fleming is due to its activity as an enzyme [EC 3.2.1.17] that hydrolyzes glycosidic bonds between the monosaccharide units of bacterial glycoprotein cell wall. Lysozyme occurs in orthologous forms in many different organisms. One of the best-studied forms occurs in relatively high amounts in hen egg white (often abbreviated HEWL). Another quite well-studied form is T4 lysozyme, from T4 bacteriophage. It performs the same catalytic function as avian and other lysozymes from higher organisms, exhibits similar structural and binding properties, but has no detectable sequence similarity. There is just enough structural similarity between HEWL and T4 lysozyme to suggest that both proteins are evolutionarily related through a distant common ancestor. Lysozyme functions as an antibacterial agent, and in some cases plays a role in digestion.
Lysozyme provides a good example of how the structural studies of enzymes using X-ray crystallography yield indispensable information about the mechanistic features of enzymatic activity. In fact, lysozyme was the first enzyme whose structure was determined crystallographically, this feat having been accomplished in the laboratory of D.C. Phillips by 1965. Lysozyme was by no means an ideal target for enzymological investigations because of the complexity of its substrate. Nonetheless, the structural information obtained by Phillips and coworkers was sufficient to allow them to propose a plausible model for the catalytic mechanism. Research since that time has largely validated their model, although new complexities and remaining unanswered questions color our current perspectives on this archetypical example of structure-function studies of enzymes.
The structure of lysozyme shows an overall globular shape, largely composed of α helix, with 15% β sheet. The helices are stacked on either side of a single, twisted antiparallel β sheet, with the latter forming an important part of a cleft that runs across an entire face of the molecule. This cleft constitutes the binding site for the substrate glycan chain.
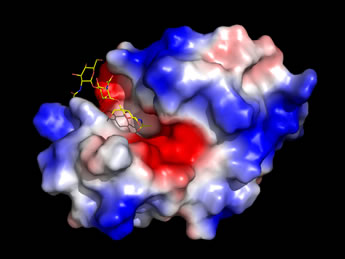
Lysozyme: Substrate & specificity
The cell walls of bacteria are composed of a highly crosslinked peptidoglycan that is composed of long polysaccharide chains that are linked by pentaglycine bridges and tetrapeptides with D-amino acid residues. The repeating unit of the polysaccharide chains is an N-acetylglucosamine, N-acetylmuramic acid disaccharide. In the crosslinks, the lactyl side chain of N-acetylmuramic acid (NAM) forms an amide linkage with a tetrapeptide. The O-glycosidic linkages between N-acetylglucosamine (NAG) and NAM are all of the β(1→4) type that link the C1 and C4 atoms of the sugars. Lysozyme specifically hydrolyzes the glycosidic bond between C-1 of NAM and C-4 of NAG, although it shows hydrolytic activity toward other similar substrates.
The key active site residues are Glu35 and Asp52, while Trp62, Trp63, Asp101, and Trp108 are involved in substrate binding. These residues line the cleft crossing the surface and yet are well separated in the sequence. As suggested by the figure above, this binding site cleft is able to accommodate more than three glycan residues, and indeed the enzyme contacts a six-residue stretch of substrate glycan chains that are composed of six or more monosaccharide residues. The extensive substrate-enzyme interactions contribute to binding energy that readily promotes the formation of the enzyme-substrate (ES) complex.
Lysozyme: Catalytic mechanism
As lysozyme was the first enzyme for which a structure was obtained by X-ray crystallography, it also provided the first opportunity to use the structure of an enzyme to guide proposal of a mechanism for its catalytic function.
The key residues Asp52 and Glu35 play two distinct roles in the catalytic mechanism of lysozyme. Asp52 is in a relatively polar environment, and has a normal pKa. Its negative charge would stabilize positive charge that develops if the reaction proceeds through the oxonium ion intermediate. In contrast, the γ-carboxyl group of Glu35 is in a more nonpolar environment, and has an anomalously high pKa (its pKa is thought to be about 6.5), keeping it more in the protonated form. The neutral, unionized form of Glu35 donates a hydrogen ion to the oxygen of the glycosidic linkage, assisting the breaking of the bond between sugar residues. Glu35 would not be so effective in this role if its pKa was normal.
Model building by Phillips and coworkers strongly suggested that the large cleft on the lysozyme surface could accommodate six sugar residues of a NAG-NAM alternating copolymer, but only if one of the sugar rings is distorted from the more favored chair conformation toward a half-chair conformation. The six residues, designated A through F, are cleaved between rings D and E, which is right at the location of Glu35 and Asp52, and it is the D ring that must bind in the distorted conformation. This led to the proposal for a mechanism featuring an oxonium ion intermediate, since the latter must adopt a planar arrangement of ring atoms that in turn forces the ring into a half-chair conformation.
See the lysozyme topics page for full discussion.
Proteases - proteolytic enzymes
Proteases make use of two distinct approaches to catalysis of peptide bond hydrolysis. One approach is to bind substrate water in proximity to the scissile bond and activate it to be a better nucleophile. This is the means employed by metalloproteases and aspartyl proteases. A second approach is based on a covalent catalytic strategy uses the enhanced nucleophilic character of an amino acid functional group to cleave the scissile bond, with water subsequently adding to a reactive intermediate. This is the approach of the cysteine proteases and the serine proteases, in which a Cys or Ser residue act as nucleophiles and form covalent intermediates with their peptide substrates.
Serine proteases
The serine proteases are a class of proteolytic enzymes that catalyze the hydrolysis of peptide bonds. Numerous principles are illuminated by a careful study of the general serine protease mechanism: Enhanced nucleophilicity of a functional group of an amino acid chain, covalent catalysis, general-acid and general base catalysis, structural basis of substrate specificity, and convergent evolution of a useful arrangement of active site residues - just to name the more prominent ones.
See the serine proteases and catalytic triad topics pages.
A zymogen is a precursor form of an enzyme, typically inactive or of very low activity, that is activated by processing of the zymogen to a mature active form. The processing entails specific peptide bond cleavage by a protease. The pancreatic serine proteases are an example of this means of controlling enzyme activity so that their active forms are generated in the appropriate biological or physiological context. The zymogen form of trypsin, trypsinogen, is activated as it is being secreted into the duodenum by very small amounts enteropeptidase. itself a serine protease. Active trypsin then acts on trypsinogen and other zymogens (such as chymotrypsinogen) in an amplified stage of zymogen activation. The blood coagulation cascade provides a more elaborate example of a series of zymogen activation stages, the triggering of which is highly regulated.
Further explorations of enzyme mechanisms, regulation and kinetics
The relationship between structure and function is a constant theme in biochemistry, and nowhere is this more vivid than in the case of enzymes, whose structures give us a wealth of detailed information upon which proposed mechanisms, regulatory features, and inhibitor design can be based.
Carbonic anhydrase
PyMOL scripts |
![]() |
![]() |
||||
Lysozyme PyMOL scripts
|
![]() |
|||||
Serine proteases PyMOL scripts |
![]() |
![]() |
||||
|
![]() |
![]() |
||||
Related topics pages: