BIOCHEMISTRY TOPICS
Regulation of metabolism
Regulation of glycolysis and gluconeogenesis. Regulation of the citric acid cycle and oxidative phosphorylation. Substrate cycles.
Let us consider the regulation of metabolism, and working from some of the general principles of the control of metabolic flux, we'll see how these apply to the regulatory mechanisms of central metabolic pathways. Like metabolism itself, regulation of metabolism should be viewed in terms of a network, or a broadly distributed set of influences on the rates and directions of a great number of reactions and processes, although we have a tendency to emphasize certain nodes of the network to be key loci of control.
Metabolic flux. The flux through a pathway is defined as a net rate
J = vf − vr
which represents a flow of material through a metabolic pathway. For example, a muscle cell carrying out glycolysis under fully aerobic conditions would have a positive flux of glucose 6-phosphate through to the end product pyruvate. The glycolytic flux presumably would correspond to the rate of conversion of phosphoenolpyruvate (PEP) to pyruvate at the pyruvate kinase step minus the rate of conversion of pyruvate to PEP (via pyruvate carboxylase and PEP-carboxykinase steps). Furthermore, we can imagine flux being measured at any given time at various steps of glycolysis. If the muscle cell carries out glycolysis under a steady-state condition, then J is constant with time, and the net rates of the individual steps of glycolysis would be proportionately correlated. For example, the flux J from glucose 6-phosphate to fructose 6-phosphate would be matched by flux from fructose 6-phosphate to fructose 1,6-bisphosphate.
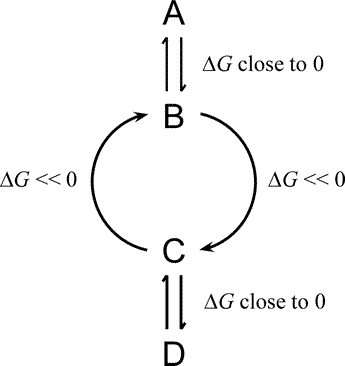
The regulation of the glycolytic pathway is coordinated with that of its alter-ego pathway, gluconeogenesis, as well as with glycogen metabolism.
Control of glycolysis and allosteric regulation of PFK
Control of glycolysis illustrates a key principle: the flux through a pathway is regulated at steps that are associated with a significant drop in free energy, i.e. at exergonic steps. The first significantly exergonic step that is unique to a given pathway is referred as the committed step of that pathway, and the committed step would seem to be a logical choice for regulation of the flux through that pathway. The most important point of control of glycolysis is held to be at the reaction catalyzed by phosphofructokinase [PFK, EC 2.7.1.1]. The reaction catalyzed by PFK is the committed step of glycolysis, and indeed PFK displays allosteric regulation. In general, PFK activity is regulated by energy charge: As a indicator of relatively high energy status, ATP acts as an inhibitor (as well as a substrate!) of PFK, while AMP (signaling low energy status), activates the enzyme. Furthermore, fructose 2,6-bisphosphate relieves the inhibitory effect of ATP, a regulatory mechanism important in liver. Levels of fructose 2,6-bisphosphate are controlled by covalent modification (phosphorylation and dephosphorylation) of a bifunctional enzyme responsible for its production as well as its breakdown.
Other regulatory features: Hexokinase and pyruvate kinase. Feed-forward activation by FBP; product inhibition by ATP. Cellular uptake by transporters. Glycolysis vs. gluconeogenesis; Substrate cycles.
Substrate cycles
If the rates of two directly opposing reactions, the phosphofructokinase reaction of glycolysis and that of the fructose 1,6-bisphosphatase reaction of gluconeogenesis are measured (with the help, say, of isotope-labeled substrates) it is found that both reactions can and do take place at the same time. Since the net result of this substrate cycle is simply the hydrolysis of ATP, it was believed at one time that this was a wasteful "futile cycle". Subsequently, it was discovered that substrate cycles can provide an effective means to create large changes in metabolic flux.
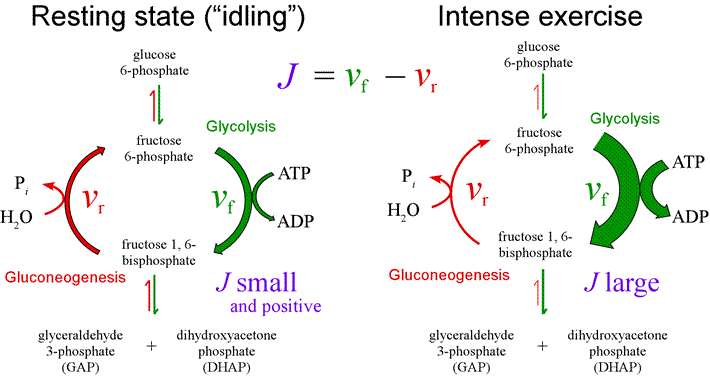
Regulation of the citric acid cycle
Most of the regulation of the citric acid cycle can be accounted for by three main influences: substrate availability, product inhibition, and competitive feedback inhibition. The rate of citrate production is effectively under the control of oxaloacetate levels. NADH, which is a product of several different steps of the citric acid cycle competes with the substrate NAD+. This product inhibition slows the rate of the citric acid cycle when the electron transport chain is unable to reoxidize NADH sufficiently. ATP, a broadly acting allosteric effector, inhibits the same citric acid cycle components subject to product inhibition by NADH, namely isocitrate dehydrogenase (IDH) and α-ketoglutarate dehydrogenase complex, as well as PDH complex. Both α-keto acid dehydrogenase complexes are also inhibited by high levels of their cognate thioester products (acetyl CoA and succinyl CoA). ADP is apparently an allosteric activator of IDH.
Regulation of oxidative phoshorylation
The regulation of oxidative phoshorylation is based upon the demand for ATP. Furthermore, the electron transport chain and phosphorylation of ADP are closely coupled, at least in the typical physiological circumstance. The rate of oxygen consumption by isolated mitochondria slows as the supply of ADP is exhausted.
Related topics pages:
- regulation of glycolysis, gluconeogenesis, and glycogen metabolism.