Lecture 36. ATP synthase
Friday 9 December 2016
Thee chemiosmotic hypothesis. The binding change mechanism. Using proton flow to drive ATP synthesis: The Berg-Oster model. P/O ratos. Uncouplers.
Reading: VVP4e - Ch.18, pp.607-614.
Summary
The process of coupled metabolic oxidation and electron transfer to oxygen with phosphorylation of ADP is termed oxidative phosphorylation. Electron transfer, occuring via the electron transport chain drives the creation of a transmembrane difference in pH. The transduction of the free energy of this transmembrane electrochemical gradient back into chemical potential energy (embodied in ATP ) is carried out by the ATP synthase complex. The many separate polypeptide chains making up this complex are organized into two major groups. A peripheral assembly with five types of subunits with the stoichiometry α3β3γδε carries out ATP synthesis. This is the F1 assembly. An integral membrane assembly called Fo functions as a proton channel.
Structural studies of ATP synthase
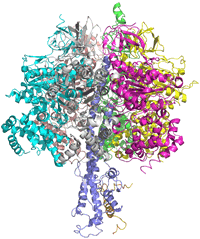
Selected structures of ATP synthase:
2wss -
3.2 Å structure of F1 portion of bovine ATP synthase
1ohh - bovine F1 ATP synthase complexed with inhibitor protein IF1, 2.8 Å
Left: Image of a structural model of the F1 portion of the bovine ATP synthase, shown in ribbon form. The α subunits are colored green, cyan, and magenta; the β subunits are yellow, salmon, and gray; the γ subunit is colored purple.
Binding change mechanism
At the heart of this proposal is the ability of the three β (beta) subunits of the F1 portion of ATP synthase complex to adopt three functionally distinct conformations. The "O" conformation ("open") has very low affinity for the adenine nucleotide substrates (ATP or ADP). The "L" conformation ("loose") has moderate affinity for substrates, but (like the O conformation) is catalytically inactive. Finally, the "T" conformation ("tight") has high substrate affinity and is catalytically active. The catalytic activity of the β subunit is of course the reaction ADP + Pi = ATP + H2O

The figure above is an abstract representation of the three β subunits of the F1 part of ATP synthase. At every step of the binding change model, each the three beta subunits is in a distinct conformation. Thus although the β subunits are intrinsically equivalent (they are all the same polypeptide chain, and have same general structure) they do not have the same conformation in the vicinity of the active site, and in fact are constrained to be different from one another in the context of the intact ATP synthase holoenzyme. Following the sequence of events in the figure above from left to right, we see that at far left the subunit in the T conformation has a tightly-bound ATP, there is a subunit in the open (O) conformation, available to bind substrate, and finally a loose (L) subunit with bound ADP + Pi. Addition of ADP + Pi, in the first step in the sequence above, leads to its association with the available catalytic site of the subunit in the O conformation. The bound ADP + Pi in the L conformer remains, but no reaction occurs, as the equilibrium ratio of [ATP]/[ADP][Pi] remains much as it is in when free in solution. Now, in the next step, proton flux through the holoenzyme complex induces a conformational change. (We ought to consider how proton flux might be coupled to a conformational change, but for now let us accept that it occurs.) The conformational change is triggered as proton flux drives a 120° rotation of the γ subunit. This in turn converts the conformation of each of the β subunits. The subunit that previously had the T conformation with bound ATP changes to the O form (thus releasing ATP - this key step of ATP release is what distinguishes the behavior of the isolated F1 from that of the holoenzyme in the presence of a transmembrane proton gradient). Simultaneously, the subunit that had the L form is driven to adopt the T conformation, and the subunit in the O conformation is converted to the L form. The newly adopted T state conformation "squeezes" ADP + Pi together making ATP formation more favorable. It is the counterclockwise rotation of the γ subunit that thus "catalyzes" the formation of ATP, and the term "rotational catalysis" is a reference to this feature. Once ATP is released from the subunit in the O conformation, ADP + Pi can then bind the vacated site, and the same sequence can be repeated with the next 120° step of the γ subunit.
The Berg-Oster model: Using proton flow to drive ATP synthesis
Clearly, the energy to drive the sequence of conformational changes that result in the synthesis of ATP according to the binding change mechanism must come from the movement of protons down the transmembrane electrochemical gradient. How exactly does the F0 proton-conducting component of ATP synthase couple proton movement to ATP synthesis? The Berg-Oster model is an elegant hypothesis to answer just this question.
Unfortunately, there is less structural information for the F0 part of ATP synthase than for the F1 part. The structure of an isolated c subunit has been determined by both X-ray crystallography and NMR. It consists simply of two antiparallel α-helices connected by a hairpin loop. The helices traverse the membrane in their native context as part of the symmetric cylindrical arrangement forming the c ring. In the middle of one of the helices is a residue - Asp41 in the F0 component of E. coli ATP synthase - which is critical for the function of the synthase. The structure of the a protein of F0 is unknown, but as stated in our text, the available evidence suggests that it possesses two hydrophilic half-channels, one leading from the cytosolic side of the membrane - the cytosolic half channel - and another leading from the matrix side of the membrane - the matrix half-channel. These half-channels are thought to lie adjacent to one another in the a subunit, but there is no connection between them, meaning that there is no direct path for protons to pass from one side of the membrane to the other.
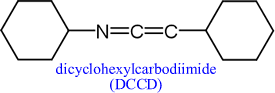
The critical role of a conserved carboxylate residue in the center of the c subunit (Asp41 in E. coli) is shown by the inactivation of ATP synthase by DCCD. This reactive compound targets carboxyl groups, crosslinking them with amines to form an isopeptide linkage. The modification of this residue prevents proton c-subunit mediated proton movement through F0.