BIOCHEMISTRY TOPICS
Gluconeogenesis
Overview of gluconeogenesis. The individual reactions of gluconeogenesis and the enzymes that catalyze them. Steps of the pathway distinct from glycolytic reactions and enzymes. Key concepts.
The process by which glucose is generated from non-carbohydrate precursors is called gluconeogenesis. Loosely speaking, gluconeogenesis is the reverse of glycolysis; but in fact, while gluconeogenesis utilizes some of the same reactions and enzymes of glycolysis, it must circumvent three essentially irreversible steps of glycolysis by alternative reactions that are more energetically favorable. The glycolytic reaction catalyzed by pyruvate kinase the final such irreversible step, is bypassed in gluconeogenesis by a two-step process. First, pyruvate must be carboxylated to form oxaloacetate, a reaction that is driven at the expense of a high-energy phosphate bond of ATP and is catalyzed by the enzyme pyruvate carboxylase. The next step - conversion of oxaloacetate to phosphoenolpyruvate (PEP) - is also an energy-requiring one, this time utilizing the high-energy phosphate bond of GTP and catalyzed by phosphenolpyruvate carboxykinase. The net reaction for the combination of the two steps is:
pyruvate + ATP + GTP + H2O → PEP + ADP + GDP + Pi + 2 H+
This reaction has a ΔG°′ (biochemical standard state free energy change) of +0.2 kcal/mol. The next reaction specific to gluconeogenesis is the hydrolysis of fructose 1,6-bisphosphate to fructose 6-phosphate catalyzed by fructose 1,6-bisphosphatase [FBPase, EC 3.1.3.11]. Finally, another dephosphorylation reaction converts glucose 6-phosphate to glucose. This hydrolytic reaction is catalyzed by glucose 6-phosphatase [EC 3.1.3.9]. In human biochemistry, glucose 6-phosphatase is mainly an enzyme of the liver, involved in the regulation of blood glucose levels.
The gluconeogenic pathway

The gluconeogenic pathway is shown, from bottom to top, in the diagram at right. The three steps distinct from those in glycolysis are enlosed by the red boxes. There are several points of entry of non-carbohydrate precursors of glucose. Glycerol, derived mainly from triacylglycerides, is converted to dihydroxyacetone phosphate. Alanine can undergo transamination to form pyruvate. Metabolites that can give rise to glucose are termed glucogenic.
Why are there distinct gluconeogenic reactions and enzymes that catalyze them? The answer is a thermodynamic one. Under normal cellular conditions, glycolysis is thermodynamically favorable, having a net ΔG of about −20 kcal/mol. Any reaction or series of reactions cannot be simultaneously favorable in both the forward and reverse directions. This would violate thermodynamic laws, since ΔG is a state function. Thus, the three major exergonic, irreversible steps of glycolysis - the reactions catalyzed by hexokinase, phosphofructokinase, and pyruvate kinase - are bypassed by distinct reactions that are themselves exergonic in the gluconeogenic direction. This is quite easy to see in the case of hexokinase and phosphofructokinase, since both of these reactions are made exergonic in the glycolytic direction because they involve the hydrolysis of ATP. The gluconeogenic reactions can be different and exergonic in the gluconeogenic direction by simple hydrolysis of phosphate esters.
Key concepts:
¶ Gluconeogenesis is formally the reverse of glycolysis, but the three exergonic steps of glycolysis must be bypassed.
¶ Low blood glucose stimulates gluconeogenesis, which takes place mainly in the liver, and to some extent in the kidney. Under these conditions, glycolytic flux is decreased. Gluconeogenesis and glycolysis are thus reciprocally regulated.
¶ Pyruvate is converted to phosphoenolpyruvate (PEP) in a two-step process via oxaloacetate (OA), which can be viewed as an "activated" form of pyruvate. Bicarbonate and the cofactor biotin are involved in this activation, which requires the expenditure of ATP.
¶ The other two distinct steps of gluconeogenesis are hydrolytic
reactions.
¶ Gluconeogenesis requires metabolite transport between mitochondria and
cytosol.
¶ Glucogenic metabolites include lactate, glycerol, citric acid cycle intermediates, and most amino acids. In animals, glucose cannot be generated from acetyl CoA and fatty acids.
¶ Substrate cycles (opposing reactions) allow large changes in net metabolic flux and are thermogenic.
Conversion of pyruvate to phosphoenolpyruvate (PEP)
The bypassing of the glycolytic step catalyzed by pyruvate kinase is actually accomplished in a two-step process catalyzed by two separate enzymes, pyruvate carboxylase and phosphoenolpyruvate carboxykinase (PEPCK). Oxaloacetate (OA) is the common metabolite of these enzymes, the product of the former and substrate of the latter in gluconeogenesis.
I. pyruvate carboxylase
Pyruvate carboxylase [EC 6.4.1.1] is a mitochondrial enzyme that is a homotetramer of 120kD subunits, each carrying a covalently-linked biotin prosthetic group. Pyruvate carboxylase is a member of a group of biotin-dependent carboxylase enzymes (also discussed below), which also includes acetyl CoA carboxylase [EC 6.4.1.2] and propionyl CoA carboxylase [EC 6.4.1.3]. The latter two enzymes play important roles in fatty acid metabolism. All the biotin-dependent carboxylase reactions require ATP hydrolysis as well as participation of the biotin prosthetic group. The general reaction catalyzed by the biotin-dependent carboxylases is shown below. The substrates are an α-keto acid (pyruvate) and thioesters. The pyruvate carboxylase reaction, for example, is represented when X = COO− and R = H.

The significance of biotin is that it acts as a carrier of CO2, which is activated to be transferred to pyruvate in the carboxylation reaction catalyzed by pyruvate carboxylase. Note that biotin is covalently attached to the enzyme via an amide bond with a lysine side chain, which creates a rather long (14 Å), flexible linker "arm".
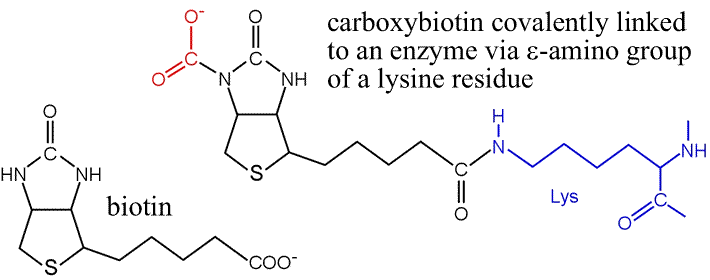
The biotin-dependent carboxylases utilize a common mechanism to generate carboxybiotin. The substrate for carboxylation of biotin is actually bicarbonate, rather than CO2. The first step in the mechanism is a nucleophilic attack by oxygen from bicarbonate on the γ phosphorous of ATP, yielding the intermediate carboxyphosphate. This intermediate is activated to transfer CO2 to biotin, forming carboxybiotin.
Pyruvate carboxylase has a dual metabolic role. When citric acid cycle intermediates are utilized as biosynthetic precursors (for example, succinate is a precursor for heme biosynthesis), the amount of OA will no longer be sufficient to match the input of acetyl CoA. Thus, in generating OA from pyruvate, pyruvate carboxylase catalyzes an anaplerotic reaction. In this context, it makes sense that pyruvate carboxylase is allosterically activated by acetyl CoA, since excess acetyl CoA signals the need for more OA. Of course, the OA generated is also an obligatory intermediate in the first stage of gluconeogenesis, the conversion of pyruvate to PEP.
Energetics and mechanism of pyruvate carboxylase
Recall that the pyruvate kinase reaction of glycolysis produces ATP and is exergonic. This tells us that the gluconeogenic conversion of pyruvate to PEP will require the input of a significant amount of energy. In accordance with this expectation, the pyruvate carboxylase reaction, which is the first step in this conversion, requires ATP.
How does pyruvate carboxylase work? The mechanism consists of two stages, each of which occurs at a different subsite of the enzyme: (i) formation of carboxybiotin, described above, and (ii) transfer of activated CO2 from carboxybiotin to pyruvate. The first stage occurs at the ATP-bicarbonate site, where formation of caboxybiotin occurs via a carboxyphosphate intermediate. This step is thought to involve release of CO2 from carboxyphosphate, followed by nucleophilic attack of the N1 nitrogen of biotin on the CO2 carbon atom. The second stage occurs when the carboxybiotin swings - about the long linker arm - into the pyruvate subsite, where it carboxylates pyruvate at C3. In this stage, the biotin cofactor apparently functions not only as a source of CO2, but also assists in generation of the enolate form of pyruvate.
II. Phosphoenolpyruvate carboxykinase (PEPCK)
Oxaloacetate is diverted to gluconeogenesis by PEPCK in a reaction that uses GTP as the donor of the phosphate that ends up in PEP, and eliminates the CO2 that was incorporated . The PEPCK reaction is therefore the decarboxylation of a β-keto acid coupled to a phosphoryl transfer to the enolate oxygen that would be the expected intermediate of this type of decarboxylation. Energetically, the favorable decarboxylation helps drive the formation of the enol phosphate, which has a significantly higher standard free energy of hydrolysis than the phosphoanhydride bonds of ATP or GTP. Furthermore, we see that the conversion of pyruvate to PEP consumes two high energy phosphate bonds.
PEPCK is a 74 kD monomeric enzyme whose subcellular localization varies with species. In some species, it is mitochondrial, and in others it is cytosolic, while in still others (notably including humans) it is roughly equally distributed in both locations. This pattern bears on the transport of metabolites across the inner mitochondrial membrane that is required for gluconeogenesis (see §16.3.3, p.454, BTS).