BIOCHEMISTRY TOPICS
Citric acid cycle
The reactions and enzymes of the citric acid cycle.
The citric acid cycle is a means of oxidation of the acetyl group of acetyl CoA to two molecules of carbon dioxide. In the complete oxidation of by oxygen, to water and carbon dioxide, the cycle works in concert with glycolysis and the pyruvate dehydrogenase complex - which represents input of acetyl CoA - and in turn produces reduced electron carriers that feed into the electron transport chain which drives oxidative phosphorylation.
Citrate synthase
The enzyme citrate synthase [EC 2.3.3.1] catalyzes the condensation of acetyl CoA and oxaloacetate. It is convenient to consider this joining of a two-carbon unit with a four-carbon unit, as the starting point of the citric acid cycle. The overall reaction occurs in two distinct steps. The first is the aldol condensation of acetyl CoA (2 C) with the α-keto dicarboxylic acid oxaloacetate (4 C) - an acylation by the thioester acetyl CoA of the α-keto group of oxaloacetate - forming citryl CoA. The following step is the hydrolysis of the latter to citrate and free coenzyme A. Both reactions are catalyzed by citrate synthase. This enzyme requires the initial binding of oxaloacetate, which causes a conformational change permitting the binding of the second substrate, acetyl CoA. The enzyme is also allosterically inhibited by ATP limiting the flux of the citric acid cycle when energy supplies are already high.
Formally, the reaction is a mixed aldol-Claisen ester condensation. Mechanistically, the reaction would be expected to occur by nucleophilic attack of the enolate of the thioester on the α-carbon (at the carbonyl group) of oxaloacetate. The hydrolysis of the thioester (citryl-CoA) that is formed in the aldol condensation step provides a significant free energy reduction (ΔG°′ = −31.5 kJ/mol), helping to drive the net reaction forward. The reaction and its stereochemistry, along with the essential mechanistic idea, is illustrated in the figure below.
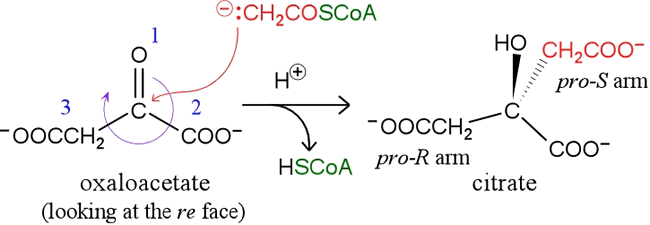
Aconitase
Aconitate is a six-carbon compound with three carboxylate groups that is derived from the dehydration of citrate. Aconitase [EC 4.2.1.3] catalyzes the isomerization of citrate to isocitrate. The isomers are interconverted by the exchange of a hydroxyl group with a hydrogen on adjacent carbon atoms. The enzyme accomplishes this through a dehydration step, producing the intermediate aconitate, followed by rehydration. Aconitase contains a 4Fe-4S iron-sulfur cluster that participates in the catalysis of dehydration/hydration reactions. (link to aconitase on Roche Biochemical Pathways map)
Isocitrate dehydrogenase
Isocitrate dehydogenase [IDH, EC 1.1.1.41] catalyzes the oxidative decarboxylation of isocitrate to α-ketoglutarate. The loss of CO2 from isocitrate occurs at the carboxyl group that is β (beta) to the keto group. Accordingly, a mechanism involving a first oxidative step to an intermediate β-keto acid, oxalosuccinate, followed by the relatively facile β-keto acid decarboxylation to the product α-ketoglutarate is probable. Direct evidence for the oxalosuccinate intermediate was obtained for an IDH variant in which the decarboxylation step was slowed to the point that it could be visualized by a fast X-ray crystallographic method (polychromatic Laue crystallography - see reference below - Bolduc, et al. 1995). The first oxidative step is coupled to the reduction of the nicotinamide cofactor (NAD+ or NADP+). There are two IDH isozymes in mammals, due to metabolic segregation of NAD+-linked oxidations and NADPH-linked reductions. One isoform, the NAD+-linked form [EC 1.1.1.42], is strictly mitochondrial. The other IDH isoform is linked to NADPH utilization, and occurs in mitochondria and cytosol. (link to IDH on Roche Biochemical Pathways map)
α-ketoglutarate dehydrogenase complex
Like PDH complex, α-ketoglutarate dehydrogenase complex consists of multiple copies of three different enzymes - E1, E2, and E3 - compose a supramolecular structure that coordinates a four step process converting an α-keto acid to a thioester (with coenzyme A) that is one carbon atom shorter, as well as redox reactions that yield NADH. The same cofactors participate in both dehydrogenase complex reactions. In the α-ketoglutarate dehydrogenase complex, E1 is α-ketoglutarate dehydrogenase [EC 1.2.4.2] , which uses thiamine pyrophosphate (TPP) as a cofactor to decarboxylate α-ketoglutarate and transfer the remaining hydroxybutyryl fragment to the lipoamide cofactor attached to E2. This results in the formation of succinyllipoamide, equivalent to reduction of lipoamide, as becomes clear upon subsequent transfer of the succinyl residue to coenzyme A, catalyzed by E2, leaving dihydrolipoamide. Here E2 is transsuccinylase [EC 2.3.1.61] and E3, which regenerates lipoamide from dihydrolipoamide, is dihydrolipoyl dehydrogenase [EC 1.8.1.4], identical to the E3 of pyruvate dehydrogenase complex.
Succinate and succinyl CoA
Succinate is a four-carbon dicarboxylic acid that can be considered chemically as a derivative of ethane, with the replacement of hydrogen by a carboxylate group on both carbons. In the metabolic cycle, succinate is derived from α-ketoglutarate in a two-step process: first, an oxidative decarboxylation catalyzed by α-ketoglutarate dehydrogenase, yielding succinyl-CoA (a thioester derivative of succinate with coenzyme A ), CO2, and NADH; second, a hydrolysis of the thioester giving succinate, coupled to generation of a hgh energy phosphate bond in the form of GTP. This reaction is catalyzed by succinyl CoA synthetase. Succinate is then oxidized to fumarate by succinate dehydrogenase, an enzyme that uses FAD to capture the electrons derived from dehydrogenation of succinate. The coenzyme A thioester of succinate, succinyl CoA, is a starting point for biosynthesis, and a end point of the catabolic pathways for the amino acids isoleucine, methionine, and valine enter the citric acid cycle by way of succinyl-CoA. The first step in porphyrin synthesis in mammals is the condensation of succinyl-CoA and glycine, which is catalyzed by δ-aminolevulinate synthase. (link to succinate and succinyl CoA on Roche Biochemical Pathways map)
Succinyl CoA synthase
Succinyl CoA synthetase [EC 6.2.1.4] catalyzes the interconversion of succinyl CoA and succinate according to the following reaction:
succinyl CoA + Pi + GDP <--> succinate + GTP + CoA
The reaction has a biochemical standard free energy (ΔG°′) of −0.8 kcal/mole in the forward direction, which is the direction followed by the citric acid cycle. This is a substrate-level phosphorylation, in which the free energy of hydrolysis of succinyl CoA (ΔG°′ ~ 8 kcal/mole) is used to generate a high energy phosphoric anhydride bond (in GTP) from phosphate and guanosine 5′-diphosphate (GDP).
Succinate dehydrogenase
Succinate dehydrogenase [EC 1.3.5.1] catalyzes the dehydrogenation of succinate to the trans unsaturated dicarboxylate intermediate fumarate. In the process, the reduced cofactor FADH2 is generated from FAD (which in this case is covalently linked to the enzyme). The enzyme also possesses an iron-sulfur cluster that accepts electrons from FADH2 and passes them on to ubiquinone, also known as coenzyme Q (Q). As part of the succinate-Q-reductase complex, succinate dehydrogenase is unique among the enzymes of the citric acid cycle in being integrally associated with the proteins of the inner mitochondrial membrane that form the electron transport chain.
Fumarase
Fumarate, a four-carbon dicarboxylate intermediate, can be considered
chemically as a derivative of ethylene, substituted with two carboxylate
groups in a trans configuration. In the citric acid cycle,
fumarate is the product of succinate dehydrogenase (which oxidizes
the central carbon-carbon bond of succinate to a double bond, producing
FADH2 as well as fumarate). Fumarate
is then converted to L-malate by a trans
addition of H and OH - carried out by the enzyme fumarase.
[EC
4.2.1.2], which catalyzes a stereospecific addition of the elements
of water.
Malate dehydrogenase
Malate dehydrogenase [EC 1.1.1.37] catalyzes the oxidation of the α-hydroxy group of malate to a keto group, forming oxaloacetate. The enzyme concomitantly reduces NAD+ to NADH. (link to malate on Roche Biochemical Pathways map at ExPASy )
Further elaborations: The stereochemistry of the citric acid cycle and hydride transfer to NAD+.